
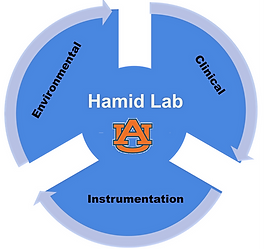
Research Projects
Rapid Discrimination of Foodborne Pathogens by Ion Mobility Mass Spectrometry
Foodborne diseases are significantly common; roughly 1 in 10 people worldwide experience illness after consuming contaminated food leading to 420,000 deaths annually as reported by World Health Organization (WHO) [1-2]. They are caused by agents that enter the body through the consumption of contaminated food or beverages followed by growth inside the host organism. They are caused by pathogenic bacteria, parasites, or viruses. Bacterial foodborne infections are currently diagnosed using the symptoms and subsequently, empirical antibiotics are being provided to patients which are usually inefficient, and they mostly lead to antibiotic resistance [3].
Bacterial food poisoning is commonly caused by pathogenic bacterial species such as Escherichia coli, Salmonella spp., Listeria monocytogenes, Staphylococcus aureus, Bacillus cereus, Clostridium perfringens, Campylobacter spp., Shigella spp., Streptococcus spp., Vibrio spp., and Yersinia spp. [2, 4]. In order to increase the recovery rates of patients suffering from foodborne infection and to reduce potential antibiotic resistance, fast robust diagnosis techniques that can work for various classes of microorganisms with high performance is required [3]. In addition, two relatively extensive studies were performed on wider sets of microorganisms with the support of advanced statistical models. The first study proved PS-MS to achieve the needed performance leading to high prediction rates for a wide set of microorganisms including multiple foodborne pathogenic bacteria. Gram-positive bacteria have been differentiated with a prediction rate of 98% utilizing negative ions solely while the sole utilization of positive ions has yielded a prediction rate of 96%, as shown in Figures 1a and 1b, respectively. Similarly, the capability to achieve high successful prediction rate was obtained for Gram-negative bacteria through data fusion statistical methodology of the positive and negative ion modes’ information which has led to a prediction rate of 87%, as shown in Figure 1c [5].
Because of the structural diversity of lipids, the addition of ion mobility separations is expected to lead to enhanced prediction rates resulting from the separation of lipid isomers. Recently, we reported the coupling of ambient ionization techniques with a commercial drift tube ion mobility mass spectrometer and demonstrated their capability for rapid separation of constitutional and geometric isomers [6]. Therefore, we are utilizing ambient ionization-IM-MS and ambient ionization-IM-MS/MS in the discrimination of closely-related microorganisms, where ambient ionization techniques include, paper spray, swab spray, and touch spray.
​
​
​
​
​
​
​
1. Quade, P.; Nsoesie, E. O., A platform for crowdsourced foodborne illness surveillance: description of users and reports. JMIR public health and surveillance 2017, 3 (3).
2. Ellis, D. I.; Muhamadali, H.; Chisanga, M.; Goodacre, R., Omics Methods For the Detection of Foodborne Pathogens. 2015.
3. Caliendo, A. M.; Gilbert, D. N.; Ginocchio, C. C.; Hanson, K. E.; May, L.; Quinn, T. C.; Tenover, F. C.; Alland, D.; Blaschke, A. J.; Bonomo, R. A., Better tests, better care: improved diagnostics for infectious diseases. Clinical Infectious Diseases 2013, 57 (suppl_3), S139-S170.
4. Quintela-Baluja, M.; Böhme, K.; Fernández-No, I. C.; Alnakip, M. E.; Caamaño, S.; Barros-Velázquez, J.; Calo-Mata, P., MALDI-TOF Mass Spectrometry, a Rapid and Reliable Method for the Identification of Bacterial Species in Food-Microbiology Laboratories. Novel Food Preservation and Microbial Assessment Techniques 2014, 353.
5. Hamid, A. M.; Jarmusch, A. K.; Pirro, V.; Pincus, D. H.; Clay, B. G.; Gervasi, G.; Cooks, R. G., Rapid discrimination of bacteria by paper spray mass spectrometry. Analytical chemistry 2014, 86 (15), 7500-7507.
6. Olajide, O. E.; Donkor, B.; Hamid, A. M., Systematic Optimization of Ambient Ionization Ion Mobility Mass Spectrometry for Rapid Separation of Isomers. Journal of the American Society for Mass Spectrometry 2021.
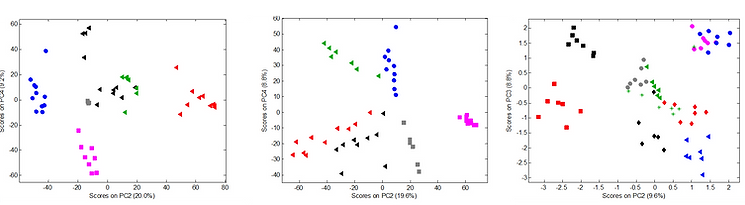
Figure 1. Principal Component Analysis of (a) Gram-positive bacteria using negative ion mode information. (b) Gram-positive bacteria using positive ion mode information. (c) Gram-negative bacteria using fused positive and negative ion modes’ information.
Direct Identification of Pesticides from fruits and vegetables
Contamination of food with pesticides can be a significant source of many serious diseases such as cancer, malformations, and damage to the endocrine, nervous and immune systems. Therefore, different communities have established maximum residue limits of pesticides, and subsequently sensitive and reliable analytical methods are required to determine the level of pesticide residues [1]. Currently, tandem mass spectrometry coupled to LC (LC-MS/MS) presents the standard method for the detection and quantitation of various pesticides’ residues [2]. It is noteworthy that the mass spectrometric and chromatographic analyses are typically performed after sample extraction and cleanup steps which are laborious and expensive steps [4-5]. In fact, the complexity in LC-MS analysis is caused by the need to transfer different analytes from matrices to vacuum as ions, while ambient ionization techniques connect the sample pretreatment and ionization into one step [3].
Utilizing Leaf Spray (LS), pesticides can be detected directly from the surface of leaves [4]. In addition, ion mobility can be used to separate isomers while computational studies can be used to identify the structures, as shown below.
​
​
​
​
​
1. Stachniuk, A.; Fornal, E., Liquid chromatography-mass spectrometry in the analysis of pesticide residues in food. Food Analytical Methods 2016, 9 (6), 1654-1665.
2. Sivaperumal, P.; Anand, P.; Riddhi, L., Rapid determination of pesticide residues in fruits and vegetables, using ultra-high-performance liquid chromatography/time-of-flight mass spectrometry. Food chemistry 2015, 168, 356-365.
3. Evard, H.; Kruve, A.; Lõhmus, R. n.; Leito, I., Paper spray ionization mass spectrometry: Study of a method for fast-screening analysis of pesticides in fruits and vegetables. Journal of Food Composition and Analysis 2015, 41, 221-225.
4. Olajide, O. E.; Donkor, B.; Hamid, A. M., Systematic Optimization of Ambient Ionization Ion Mobility Mass Spectrometry for Rapid Separation of Isomers. Journal of the American Society for Mass Spectrometry 2021.
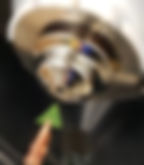



Figure 2. (Left) Schematic of LS-IM-MS, (Center) LS–IM–MS analysis of terbuthylazine and propazine pesticide isomers. (Right) Optimized structures of (A-B) terbuthylazine and (C-D) propazine with their relative energies (kcal/mol) at B3LYP/6-31G(d,p) level of theory and their corresponding collision cross section values.